Non-invasive 3D fluoroscopic/CT imaging of the Wrist
Elan Harris, BA, Pichitchai Atthakomol, MD, Julian Korteweg, MD, Guoan Li, PhD, Ali Hosseini, PhD, Amir Kachooei, MD, Neal C. Chen, MD
©2021 by The Orthopaedic Journal at Harvard Medical School
BACKGROUND Some patients who injure their wrist through a fall on an outstretched hand develop carpal instability (CI), and a subset of these patients may develop arthritis secondarily. Scapholunate interosseous ligament (SLIL) injury is the most prevalent type of CI, but there is no gold standard treatment. Surgical intervention is unable to restore original biomechanics of the wrist. Therefore, there is a need to better understand biomechanics of the proximal carpal row and specifically the scapholunate junction itself. The purpose of this study was to investigate the use of a multi-modal CT plus dual-fluoroscopy imaging system (DFIS-CT) and we hypothesized that this could serve as a lower dose alternative to 4DCT kinematic imaging, as has previously been described for knee, hip, and ankle, but not wrist.
METHODS We describe an investigational pilot study evaluating the feasibility of this DFIS-CT imaging technique utilizing a single computed tomography scan of the wrist, then creating a three-dimensional (3D) model that can be matched to biplanar, orthogonal fluoroscopic images. Through this approach, DFIS-CT produces viable dynamic models with six degrees of freedom (6DOF). Furthermore, this method can model multiple ranges of wrist motion while exposing patients to ½ - ¼ the radiation dose of a single motion series from 4DCT kinematic imaging.
RESULTS Using these techniques, we evaluated two normal wrists compared with a wrist with static scapholunate diastasis. We successfully applied DFIS-CT to demonstrate six ranges of wrist motion in each subject and were able to display differences in carpal bone configuration in the patient with SLIL injury relative to the wrists of uninjured subjects.
CONCLUSION The DFIS-CT method presents a low-dose alternative to in vivo dynamic imaging of 3D carpal kinematics that, after further elucidation, may provide a means to assess SLIL repair failure among other carpal abnormalities.
LEVEL OF EVIDENCE Level III Diagnostic Study
KEYWORDS Wrist Injury; kinematics; biomechanics; scapholunate ligament Injury; CT; dual-fluoroscopy; 3D imaging
Among patients who seek medical attention resulting from falling onto an outstretched hand, as many as 44% present with some degree of carpal instability (CI), of whom a subset will develop some degree of arthritis.1 Scapholunate interosseous ligament (SLIL) injury is the most common form of CI, especially common in young people and athletes, but there is no gold standard treatment and surgical intervention often seems to be unpredictable clinically and does not restore the native kinematics of the wrist.2-4 Therefore, there is a need to better understand biomechanics of the proximal carpal row and scapholunate articulation.
Previous studies of wrist kinematics explored motion either in vivo or in vitro. In vitro methods include tracking the motions of the wrist using a dynamic wrist simulator. This method has the advantage of being able to record real-time motion of wrist kinematics. In this manner, exhaustive six-degree-of-freedom (6DOF) analysis of carpal bone motion has been investigated. Recently, cadaveric studies have shown promise in evaluating wrist injuries such as kinematic correlations between scaphoid and lunate rotations, highlighting the tensile and torsional properties of the SLIL.3,5 These findings may provide insight into potential improvements upon surgical planning and treatment for SLIL injuries that have historically been unreliable. However, these methods are not easily interpreted. The invasive requirement for surgical embedding of radiographic markers or electromagnetic sensors within the carpal bones could interfere functionally with the capsule and ligaments of the wrist, while variability in the positioning of the implanted markers might affect the results of the studies.6-9
Four-dimensional computed tomography (4DCT) can provide in vivo dynamic imaging but has limited availability currently and due to radiation dose is constrained from assessing multiple dynamic arcs of motion. Thus, we investigated the use of a CT plus dual-fluoroscopy imaging system (DFIS-CT) as has previously been described in knee, hip, and ankle, but not in the wrist.
A dual-fluoroscopy imaging system (DFIS) can be paired with a single CT to track kinematics of joints by combining the three-dimensional (3D) accuracy of CT rendering with the ability of dual-fluoroscopy to take multiple views of joints in various ranges of motion. This hybrid technique, described and piloted in the current report, integrates the benefits of both modalities and uses dual-fluoroscopic images and CT scans as an integrated non-invasive technique to measure in vivo joint kinematics with a lower amount of radiation dosage, since only one CT scan in neutral position is required. The data are also more precise than for the 4D multi-CT method alone due to the ability to capture multiple images from the dynamic dual-fluoroscopic imaging system (DFIS) throughout the full arc of each designated motion. This technique has already been elucidated in the shoulder, hip, knee and ankle joints with high accuracy, precision, and repeatability.10-17 To the best of our knowledge, no studies have reported the use of DFIS paired with CT in tracking the kinematics in the wrist. The purpose of our study was to investigate the feasibility of our technique and we hypothesized that DFIS-CT could provide a dynamic 3D model as a lower radiation alternative to 4DCT kinematic imaging in each joint position in a variety of ranges of motion using a non-invasive in vivo hybrid imaging technique for both uninjured wrist cases and an example patient with SLIL injury. Dynamic 3D models of normal and SLIL injuries could help elucidate why surgical interventions are often unsuccessful and what differentiates successful cases from unsuccessful ones, potentially leading to a better understanding of which cases are good surgical candidates and why others are not, ultimately leading to better outcomes and a reduction of unsuccessful procedures.
This study was conducted at Massachusetts General Hospital from June 2016 - June 2017. Written informed consent was obtained in accordance with the approval of the institutional review board (IRB). Two right-dominant upper extremities participants (one male and one female, (age 27 and 35) with no previous wrist injuries, as well as one male patient (age 29) with an existing scapholunate-ligament injury were imaged using CT while in neutral forearm rotation and elbow extension (GE Light Speed Pro 16-Slice scanner; General Electric, Milwaukee, WI). Previously, our group investigated the effect of elbow position on distal radioulnar joint kinematics.18 In the current study, our group’s goal was to apply a similar approach to the more complex anatomy of the wrist and its corresponding injuries. The subjects were instructed to lay motionless with outstretched hands on a table that slides into the CT scanner. Each patient’s hand was laid flat to ensure consistency and repeatability. The duration of the CT scans was approximately 2 minutes. A CT scan was performed of the wrist in this position that included 281 axial slices with image spacing of 0.625mm with a resolution of 512 X 512 pixels and a field of view of approximately 280mm. The CT images were then marked to segment and build 3D triangular-mesh models using a Canny filter in a commercially available software package (Matlab, Mathworks, Canton, MA). The Canny filter was used to calculate gradients in pixel intensity to detect edges between objects. These calculated edges were employed in the tracing of the outlines of the carpal bones inside of the axial plane image using solid-modeling software (Rhinoceros; Robert McNeel & Associates, Seattle, WA.) In order to complete these models, these newly constructed Contours were aligned in the correct planes in a three-dimensional space, and the surface was meshed automatically using the solid-modeling software.
Once we had obtained our 3-dimensional CT image-based computer models, two orthogonally placed fluoroscopes (BV Pulsera, Philips, Eindhoven, Netherlands) were used to capture images of the wrist throughout each range of motion. Each fluoroscope produces real-time 1K x 1K image resolution and has an image intensifier diameter of 31 cm. When using the two fluoroscopes to obtain orthogonal images, the total imaging volume can reach up to 30cm x 30cm x 30cm. The system allows the investigator to capture the two orthogonal images simultaneously. Using this dual-fluoroscopy imaging system (DFIS) the wrist was imaged across each performed motion. The plane of the C-arm was placed coplanar to the floor and the subject was seated with the elbow and wrist resting on a table. Each subject was imaged at fixed, evenly-spaced time-intervals while performing fully dynamic activities including moving the wrist from extreme dorsiflexion to extreme palmar flexion (3 seconds x 11 images), moving the wrist from extreme ulnar deviation to extreme radial deviation (3 seconds x 11 images), moving the wrist and forearm from full supination to full pronation (3 seconds x 11 images), and moving the wrist as if throwing a dart from extension-radial deviation to flexion-ulnar deviation (3 seconds x 11 images) (Figure 1 and Figure 2). They were also asked to perform a tight fist and hold (3 seconds x 2 images) one image was taken while the fist was held, but relaxed and the second, after the fist was clenched, as well as leaning on a wall with an outstretched hand (3 seconds x 2 images) with one image occurring before pressure was applied and one occurring after.
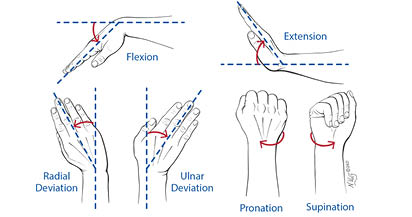
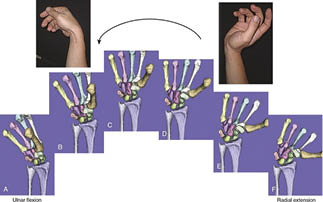
The DFIS images were then imported and registered into the solid modeling software to determine the in vivo positions of the radius, ulna, scaphoid, lunate, and triquetrum from each movement progression (Figure 3). Then, the radiographs’ silhouettes were outlined by hand in each frame at each angle to provide a guide for matching (Figure 4). The static model previously created for each participant from CT imaging was also imported into the three-dimensional modeling space and matched to each pair of fluoroscopic images by adjusting each individual bone’s x, y, and z axes in 6DOF so that its projection aligned optimally with the outline of each orthogonal radiograph (Figure 5). This was accomplished by toggling back and forth between the 2 orthogonal directions corresponding to the position of the x-ray source of the fluoroscope during image acquisition. The final results showed harmonious motion of the 3D anatomical model of each carpal bone in each arch of motion of the wrist joint. Our method was previously validated using cadaver studies in a knee model to an accuracy of 0.1 mm position and 0.1° rotation.12
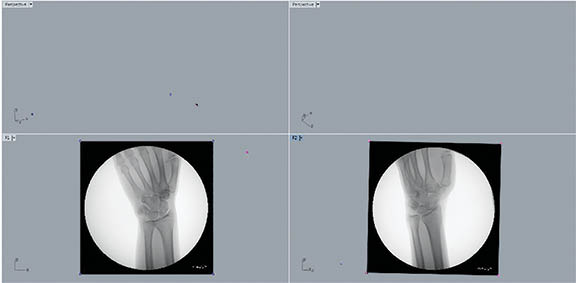
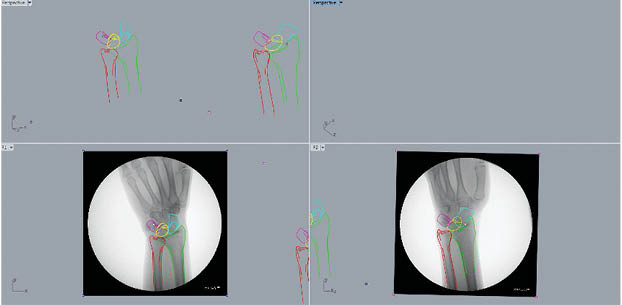
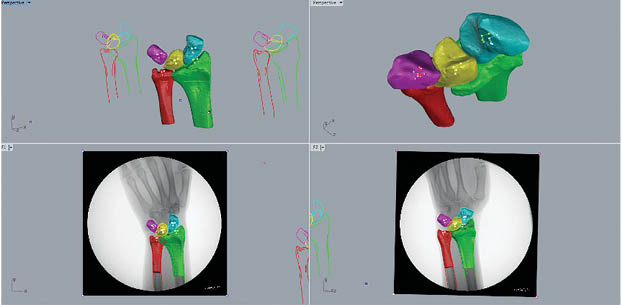
We demonstrated an example of the method used to create 3D models in each range of motion from DFIS (Figure 3, Figure 4, Figure 5, Figure 6) and examples comparing models in control subjects and an SLIL injury patient. When performing the DFIS-CT technique, our group observed normal joint kinematics using healthy subjects (Figure 6 and Table 1). We also observed the abnormal kinematics of the scapholunate ligament injured wrist that demonstrated the typical scapholunate widening, flexion-pronation of the scaphoid, as well as dorsal subluxation of the scaphoid, with extreme gapping between the scaphoid and lunate observed from multiple angles in the SLIL injury patient as compared to control (Figure 7 and Figure 8).
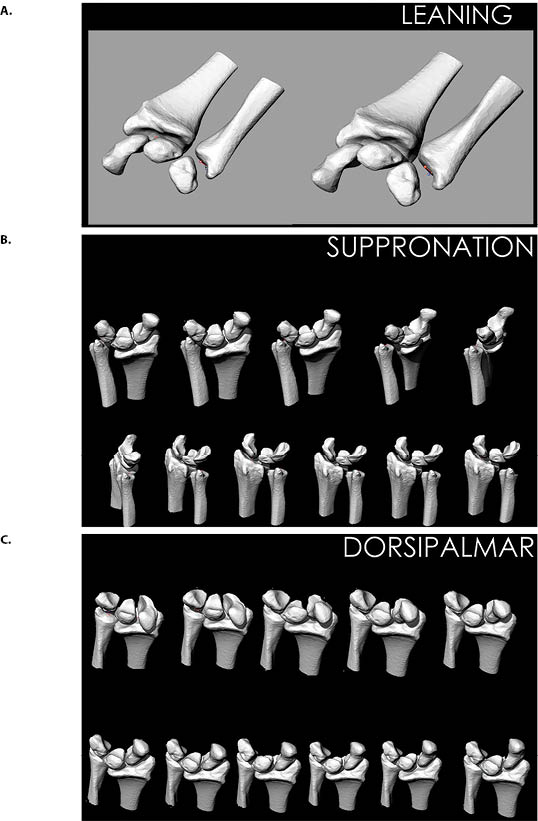
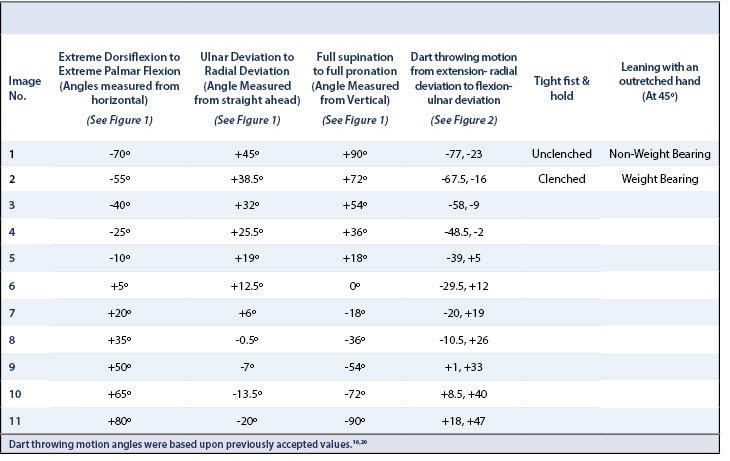
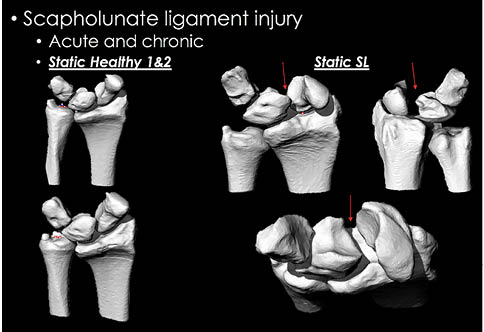
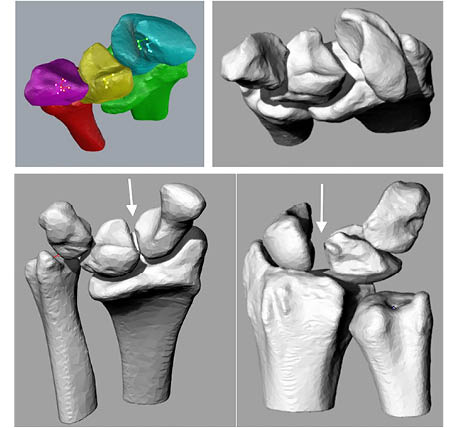
Using the DFIS-CT method, we were able to model the 3D kinematic motion of the wrist in two healthy volunteer subjects and on one patient with SLIL injury for the following wrist movements (as detailed in Table 1):19,20
- Extreme dorsiflexion to extreme palmar flexion
- Ulnar deviation to radial deviation
- Full supination to full pronation
- Tight fist and hold
- Leaning with an outstretched hand
We used a DFIS-CT hybrid technique to create 3D kinematic imaging models moving through various arcs of motion of the wrist. The benefits of this technique include the ability to scan and reconstruct 3D kinematic views for multiple full motion series while applying a limited amount of radiation dosage exposure compared to a 4D CT scan. Only one CT scan is needed in a neutral position, and DFIS generates a low dose of radiation. The total amount of radiation exposure in this study is equal to a whole-body exposure of 0.04 mSv. This amount of radiation is about the same as one would normally receive in approximately five days from natural background sources from the earth and the sky.21 Furthermore, we can create comprehensive data using this method, with the ability to capture multiple images from DFIS during each of a variety of arches of motion.
We were able to successfully segment and match each 3DCT model with each DFIS image at given angles throughout each motion in a consistent and systematic fashion (Table 1). The dynamic models created by this matching system visually demonstrate the effectiveness of this technique as each set of extracted images closely mirror those of normal wrist motion (Figure 6).
In particular, the dart throwing motion (DTM) is important because it is characterized by proximal row kinematics unique to humans and displays multiple planes of motion simultaneously, which are common in everyday activities. The dart-thrower’s motion in rehabilitation is debated. While some studies argue this is the natural axis of the midcarpal joint, Garcia-Elias et al. have found that this motion may be problematic in early rehabilitation.22-24 Dynamic 4DCT has shown that DTM directly affects the SL joint following injury and while initially this motion was thought to be a useful rehabilitation procedure, it may actually be detrimental to recovery following SLIL repair without additional joint stabilization.5,25 Using DFIS-CT coupled with cadaveric data to analyze these unique proximal row kinematics and comparing them with those of injuries like that of the SLIL may provide valuable insight into improving treatment options for such injuries.
Four-dimensional CT (4DCT) techniques, which use CT to capture multiple images over time to create an animated video, have proven useful in cardiac and pulmonary evaluation. Recently these techniques have become more widely used to investigate joint kinematics, specifically, wrist biomechanics before and after surgical repair demonstrating apparent differences between mid-carpal kinematics following scapholunate injury.26,27 Although this technology continues to advance, attempting to lower radiation exposure, each scan still delivers 0.07-0.17mSv on average, roughly 2-4x the radiation of DFIS-CT for each range of motion.26 Thus, performing 4DCT across six ranges of wrist motion would expose the patient to 12-24x the dose delivered with DFIS-CT for similar output data. Patient radiation should follow the ALARA (as low as reasonably possible) principle, therefore favoring DFIS-CT, the lower dose technique. In addition, if the hybrid technique were employed, follow-up studies using only biplanar fluoroscopy without CT scanning may be sufficient.
Just as some of the drawbacks of 4DCT include massive amounts of data, which must be analyzed, DFIS-CT analyses can also be time consuming. However, based upon current developments in image processing and analysis enhanced with machine learning and artificial intelligence (AI), there are opportunities for future improvements in the efficiency of this technique by developing AI-assisted software. For example, there are currently two time-consuming aspects of this technique that could be greatly reduced with AI. First, building the wire mesh models layer by layer can now be done using advanced imaging such as the 3D quantitative imaging (3DQI) software developed in MGH radiology (3dqi.mgh.harvard.edu/project/intro), which would be especially helpful for segmenting smaller bones like the pisiform, which we decided not to include in our analysis because it proved to be challenging. Second, the manual matching of the imported 3DCT models within the computer modeling software with orthogonal radiographs requires both extensive knowledge of carpal anatomy and practice with modeling manipulation. Even with these competencies, the process runs through multiple rounds of adjustment and readjustment for almost a hundred images per patient. We believe that by harnessing the power of AI and advanced imaging we could greatly reduce both the time and knowledge required to perform this technique, thereby making it widely accessible and superior to 4DCT for assessment of joint kinematics.
Additional limitations of the current study include the small number of subjects, and lack of a gold standard comparison to perform a quantitative validation of accuracy. In order to validate the accuracy of the combined DFIS-CT technique for human wrist kinematics, a further study should be conducted that compares the matched 3D models to in vitro data taken from cadaveric wrists. We plan to expand the current number of subjects, both injured and non-injured, and develop quantitative metrics that may guide treatment.
The demonstration of feasibility of a non-invasive 3D wrist kinematic imaging technique as described in the current study contributes important information for the development of wrist joint kinematic analysis, which could deepen our understanding of the complex movements of wrist components in normal subjects and in patients with a variety of wrist injuries and disorders at lower radiation dose to the patient than current methods. Such a technique could prove essential for assessing individual anatomy, determining likely candidates for successful surgery, and may be the key to unlocking the mystery of SLIL treatment failure and carpal instability for many patients.