Core Muscle Size and Mortality Following Non-Operative Management of Pelvic Fractures
Shaun P. Patel, MD, William D. Scheidler, MD, Sven A. Holcombe, BS, Stewart C. Wang, MD, PhD, James A. Goulet, MD
The authors report no conflict of interest related to this work.
©2016 by The Orthopaedic Journal at Harvard Medical School
INTRODUCTION The incidence of pelvic fractures in the United States is 13 cases per 10,000 person-years, of which 94% are low-energy fractures in the geriatric population. This cohort has a 20% one-year mortality rate, yet there are limited data predicting post-fracture survival. Core muscle size, a proxy measure of patient frailty, may be one such variable to help risk stratify patients.
METHODS We identified 405 patients undergoing non-operative management of a pelvic fracture who had a CT scan of their abdomen/pelvis within 90 days of fracture. We selected for fragility fractures by including females over 50 and males over 65, with an Injury Severity Score (ISS) less than 18. Core muscle size (cross-sectional area of the psoas muscles at L4, normalized to height) was correlated to post-fracture mortality.
RESULTS One-hundred and three patients met our selection criteria (82.5% female). Twenty-four patients died within one year of their diagnosis (23.3%). Patients in the lower third of normalized psoas sizes had significantly higher one-year mortality rates compared to patients in the upper third (females: 39.3% vs. 6.9%, p = 0.005; males: 100.0% vs. 0.0%, p = 0.002).
CONCLUSION In non-operatively managed pelvic fractures, our data indicate that decreased core muscle size leads to significantly higher mortality rates. Such objective measures of patient frailty may inform clinical decision-making and improve orthopaedic patient risk stratification.
Pelvic fractures are a major health care burden, especially in an increasing elderly population. The incidence of pelvic fractures in the United States alone is estimated to be 13 cases per 10,000 person-years.1,2 In 2005, the United States’ health care expenditure on pelvic fractures reached nearly 900 million dollars in direct costs alone, a value which is growing exponentially each year.3,4 A disproportionate fraction of these injuries occur in an osteoporotic population,5,6 a common comorbidity affecting over 55% of patients age 50 and older.7 Osteoporotic fractures account for 94% of fractures in patients over the age of 60.8 These injuries are typically low-impact in nature and often managed conservatively without an operation. However, they have serious consequences in an already at-risk population. One out of every five geriatric pelvic fracture patients dies within a year of their injury.9 Of the remaining, one in four patients who previously lived independently is forced to stay in a nursing home for at least one year following their pelvic fracture.7,10
While factors contributing toward the incidence of pelvic fractures are well known,10-13 there are very few described objective measures for predicting outcomes following management of these fractures. Fracture classification systems exist to determine the type of management a fracture should receive based on characteristics such as anatomy, stability, or mechanism of injury. Unfortunately, these variables are not reliable predictors of post-fracture outcomes.14 Conversely, previous research has demonstrated that frailty is independently associated with an increased risk of adverse outcomes including recurrent falls, pelvic fracture, and mortality in the elderly.15,16 Frailty has previously been defined by a constellation of relatively subjective criteria including unintentional weight loss, weakness, and self-reported lethargy.15 While the prior research suggested a correlation between frailty and pelvic fracture mortality, further evaluation is necessary to more objectively assess frailty and its relationship to outcomes following pelvic fracture.
Morphometry may provide one such objective measure of frailty. Prior morphometric research has demonstrated strong relationships between frailty, core muscle size, and outcomes in vascular and transplant patients.14,17,18 In fact, these studies revealed core muscle size, a proxy measure of patient frailty, to be the strongest independent predictor of post-operative mortality in these patient populations.14,17 However, no work has yet investigated this relationship in non-operative, orthopaedic populations.
This study aims to explore the clinical utility of core muscle size as a proxy measure of patient frailty in predicting outcomes of patients undergoing non-operative management of pelvic fragility fractures. Specifically, we hypothesize that decreased core muscle size is associated with increased mortality.
We performed a retrospective cohort analysis of 405 patients who underwent non-operative management of a pelvic fracture at a Level I trauma center between February 2004 and January 2011 and who had a CT scan of their abdomen/pelvis within 90 days of their diagnosis. We selected for a low-impact osteoporotic fragility fracture mechanism by including only females over age 50 and males over age 65 and only those with an Injury Severity Score (ISS) less than 18. Demographic, core muscle size, and post-treatment mortality data were collected for all patients included in the study.
To assess core muscle size, cross-sectional areas of the left and right psoas muscles at the level of the fourth lumbar vertebra were determined in our study population. These morphometric calculations were completed using semi-automated algorithms programmed in MATLAB R2011a (MathWorks, Natick, MA). This was accomplished by first identifying individual vertebral levels on each patient’s CT scan. We then selected the individual imaging slice at the superior aspect of the fourth lumbar vertebra (L4) and outlined the borders of the left and right psoas muscle. These outlines were performed by validated investigators who demonstrated significant intra- and inter-observer reliability in previous training sessions. The area of the resulting enclosed regions was then computed to generate the cross-sectional area of the psoas muscles (Figure 1). To account for potential body size and gender biases, total psoas area (TPA) was normalized by height (TPA divided by height) and patients were also analyzed separately by gender.
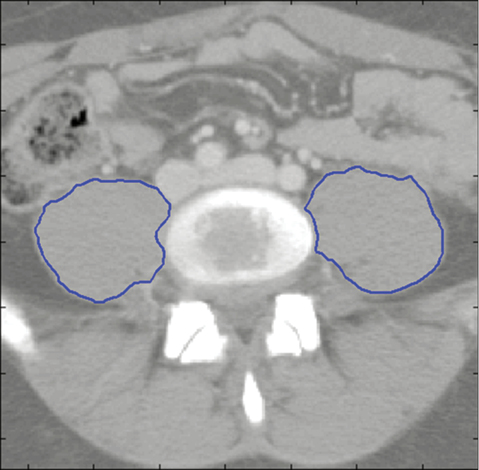
Continuous variables are reported as mean ± SD. Statistical analysis of continuous variables was accomplished using Student’s t-test. Categorical variables pertaining to the frequency of outcomes, such as mortality rates, are reported as percentages. Frequency data were evaluated by the creation of 2 x 2 contingency tables and were analyzed using Fisher’s exact test. Statistical computations were performed using StatView (SAS Institute, Inc., Cary, NC). For this study, α was set a priori at 0.05.
One-hundred and three patients met inclusion criteria for our study. Descriptive data for this population are shown in Table 1. The mean age at the time of management was 75.4 years (74.5 for females and 79.8 for males). The mean total psoas area (TPA), defined as the sum of the right side and left side psoas area, was 1333.1 mm2 for females and 1956.2 mm2 for males. Overall one-year mortality rates were 23.3% (17.6% for females, 50.0% for males).
To describe the effect of TPA on one-year post-management survival, patients in each gender were stratified into tertiles by their height-normalized TPA (Tables 2and 3). Patients in the upper TPA tertile had six-month and one-year mortality rates of 6.9% for females and 0.0% for males. Correspondingly, mortality rates for the lower TPA tertile patients were found to be 32.1% for females and 83.3% for males at six months and 39.3% for females and 100.0% for males at one year (Figures 2 - 5).
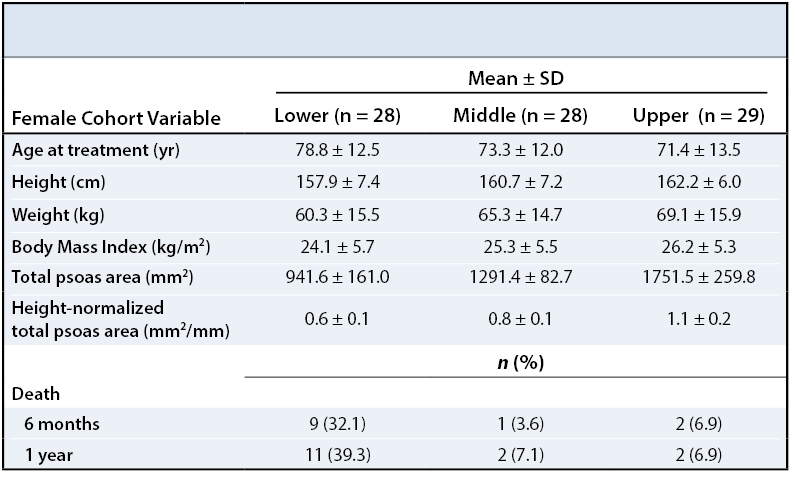
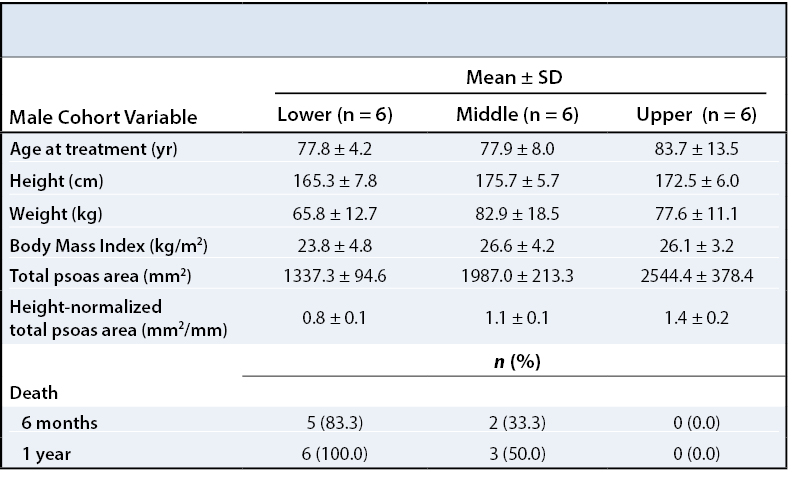
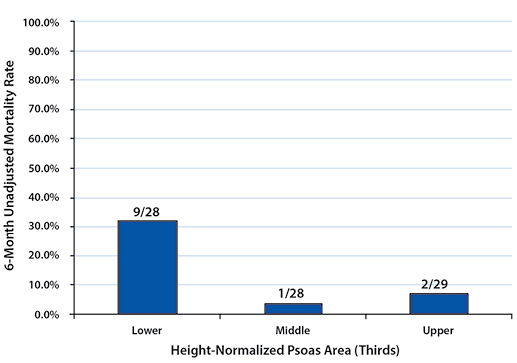
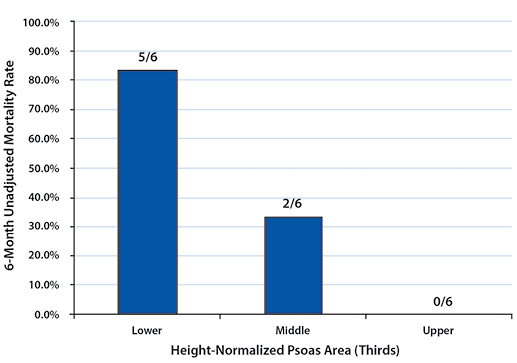
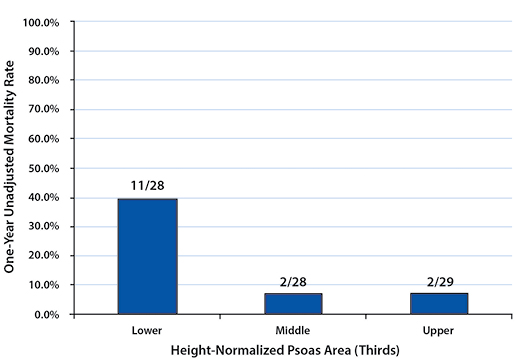
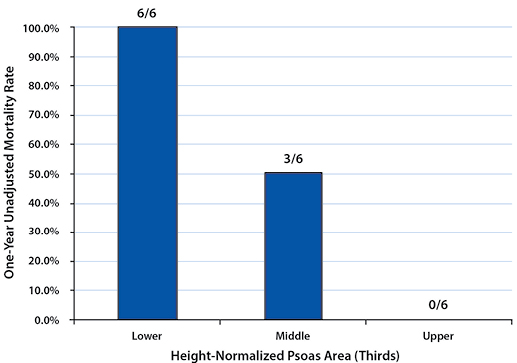
Mortality rates between the upper and lower tertiles of both females and males at six-month and one-year time points were found to be significantly higher (six-month: p=0.021 for females, p=0.015 for males; one-year: p=0.005 for females, p=0.002 for males).
There is a relative paucity of objective risk assessment and mortality studies in the elderly pelvic fracture population. Due to the high incidence and poor survival of non-operatively managed pelvic fractures, they are an appropriate starting point to evaluate the relationship between frailty and outcomes following orthopaedic procedures.8,19,20 This study utilized an objective measure of frailty, defined as core muscle size, and correlated it to mortality following non-operative management of pelvic fractures. We demonstrate a significant increase in mortality with decreased core muscle size, a trend evident across both genders. Although due to a relatively low number of overall mortalities we could not identify an absolute height-adjusted psoas size at which there is increased risk of mortality, we do demonstrate that the lowest tertile in each gender serves as a relative indicator of increased risk. Such objective measures of patient frailty may potentially inform clinical decision-making and improve orthopaedic patient risk stratification.
This study must be considered within the context of its limitations. First, this is a retrospective study at a single center with a relatively small patient cohort. The small cohort did not allow us to subdivide patients by fracture patterns, which have long been established in clinical management decisions in pelvic fracture patients. Future work should combine frailty measures with fracture patterns to best inform clinicians of patient outcomes. The small cohort also did not allow us to find absolute risk thresholds in core muscle size as compared to general populations or other fragility fracture populations. Second, there is a potential selection bias with regard to patient inclusion in the study group. The patient population was limited by the availability of viable, peri-injury CT scans. Future studies can be performed prospectively to ensure all enrolled patients have appropriate imaging. Finally, the study presumed osteoporosis due to both elevated patient age and a low-velocity mechanism of injury, without actual confirmation by bone densitometry. The diagnosis of osteoporosis by DXA or CT scan could be part of the inclusion criteria in future work.20
In this study, morphometric measures of core muscle size are introduced as potentially relevant prognostic indicators of non-operative management of pelvic fragility fractures. Smaller core muscle size when adjusted for height was shown in both genders to be significantly associated with increased mortality in this population. This trend – that frailty negatively affects outcomes in fragility fracture patients – has been documented in the orthopaedic literature, but the concept of frailty has not yet been effectively quantified.15,16 To our knowledge, this study is one of the first to objectively quantify frailty using core muscle size in the orthopaedic literature.
Quantifiable frailty measures such as core muscle size could become a very important risk-stratification tool for the geriatric orthopaedic trauma population.16 It is likely that the path to complications and mortality begins with slight deviations from the routine recovery pathway. This is compounded by a diminished physiologic buffer until a critical point is reached and an inferior outcome is inevitable. An objective measure of overall burden of frailty, such as through core muscle size, could inform novel treatment pathways in an effort to avoid these poor outcomes. Proactively measuring core muscle size and quality could even stratify patients for highly targeted nutritional and physical therapy interventions to prevent or delay further functional decline. A larger, prospective study utilizing core muscle size as a marker of frailty will be a useful adjunct to better understand the relationship between frailty and orthopaedic outcomes including not only mortality, but also morbidity and health care expenditure.
Our results suggest that MLB pitchers born in regions with colder average temperatures, who are more likely to break from throwing during the winter season, may have longer professional pitching careers as a result of less career arm injury and exhaustion. We suggest that pitching year round at an early age may be detrimental to the longevity of Major League pitchers. These findings support the need for continued pitch-count regulation in youth baseball and the need for monitored rest from throwing during the offseason.
We thank Garin G. Hecht, MD for his editorial and administrative roles on the study team.